Quantum computing is an innovative approach to computation that leverages the principles of quantum mechanics to perform complex calculations at a significantly faster rate than classical computing.
Unlike classical computers that use bits to represent information as either a 0 or 1, quantum computers use qubits, which can exist in multiple states simultaneously due to the phenomenon of superposition. This ability allows quantum computers to process vast amounts of data in parallel, leading to the potential for solving problems that are currently intractable for classical computers.
One of the key features that sets quantum computing apart is entanglement, where qubits become interconnected and their states are interdependent, regardless of the physical distance between them. This property enables quantum computers to perform calculations that would be impossible for classical computers, providing a promising avenue for tackling challenges in various fields such as cryptography, optimization, and simulation. As researchers continue to explore the capabilities of quantum computing and overcome technical hurdles, the technology holds the potential to revolutionize industries and drive innovation in ways previously unimaginable.
The Principles of Quantum Mechanics
Quantum mechanics is the fundamental theory that governs the behavior of matter and energy at the smallest scales of atoms and subatomic particles. It provides a framework for understanding the nature of particles and waves, as well as their interactions with each other. One of the key principles of quantum mechanics is the concept of superposition, which states that a particle can exist in multiple states simultaneously until it is measured or observed.
Another important principle in quantum mechanics is entanglement, where two particles become connected in such a way that the state of one particle is directly correlated to the state of the other, regardless of the distance between them. This phenomenon highlights the non-local nature of quantum mechanics, where particles can be instantaneously influenced by each other regardless of how far apart they are. These principles underlie the foundation of quantum computing, harnessing the unique properties of quantum mechanics to revolutionize computational power and capabilities.
The Difference Between Classical and Quantum Computing
Classical computing processes data using bits that are either 0s or 1s, representing distinct states in binary code. This sequential and linear approach means that classical computers operate based on a set of defined instructions one at a time. In contrast, quantum computing leverages qubits, which can exist in superposition, representing both 0 and 1 simultaneously. This characteristic enables quantum computers to perform multiple computations at once, potentially allowing for exponential speedups in certain algorithms.
Moreover, another fundamental difference lies in how classical and quantum computers handle information. Classical computers use deterministic algorithms to provide specific outputs for given inputs, ensuring precise and predictable results. On the other hand, quantum computing introduces probabilistic elements due to the inherent uncertainty resulting from superposition and entanglement of qubits. This unique feature of quantum computing challenges traditional notions of computation and opens up new possibilities for solving complex problems that are impractical for classical systems.
Quantum Bits: Understanding Qubits
Quantum bits, or qubits, are the fundamental building blocks of quantum computing. Unlike classical bits, which can only exist in one of two states (0 or 1), qubits can exist in a superposition of both states simultaneously. This unique property allows qubits to perform complex calculations in parallel, making quantum computers exponentially more powerful than classical computers for certain types of problems.
Another key aspect of qubits is their ability to exhibit entanglement, a phenomenon where the state of one qubit is intrinsically linked to the state of another, even when they are physically separated. This correlation enables qubits to work together in a coordinated manner, leading to faster computation speeds and more efficient problem-solving strategies. By harnessing the power of qubits and leveraging their quantum properties, researchers are pushing the boundaries of computing capabilities and paving the way for groundbreaking advancements in various fields.
Quantum Superposition and Entanglement
Quantum superposition is a fundamental principle of quantum mechanics that allows particles to exist in multiple states simultaneously. This means that a quantum system can be in a combination of different states until it is measured, at which point it collapses into a single state. Superposition is what enables quantum computers to perform many calculations at once, leading to their potential for exponential speedup over classical computers.
Entanglement is another remarkable phenomenon in quantum mechanics, where two or more particles become connected in such a way that the state of one particle is dependent on the state of the other, regardless of the distance between them. This interconnectedness plays a crucial role in quantum computing by enabling qubits to be correlated and work together, leading to enhanced computational power. The unique properties of superposition and entanglement are what make quantum computing a revolutionary field with the potential to solve complex problems that are currently intractable with classical computers.
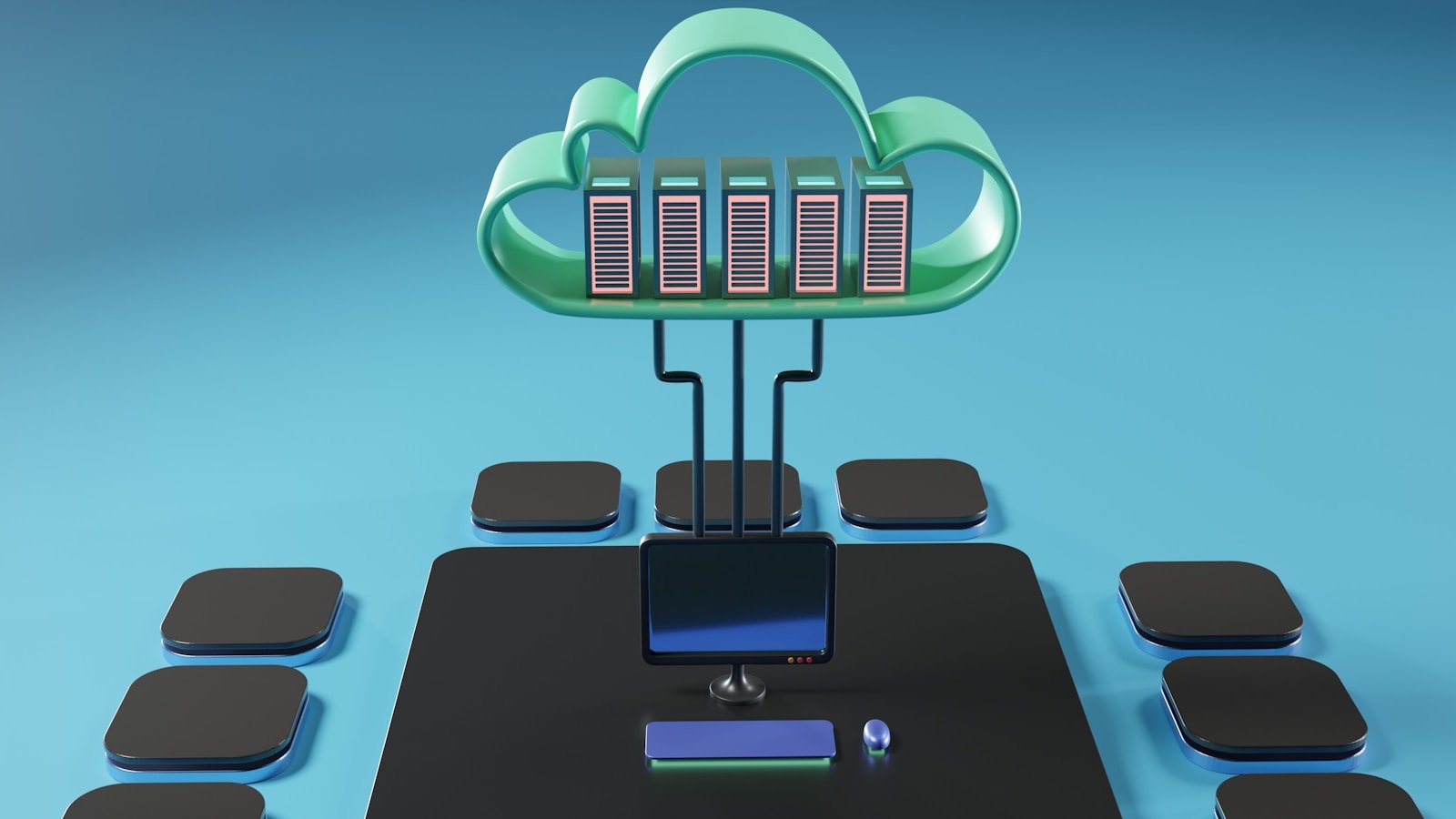
Quantum Algorithms: Shor’s Algorithm and Grover’s Algorithm
Shor’s Algorithm, developed by mathematician Peter Shor in 1994, is a quantum algorithm that poses a significant advancement in the field of cryptography. This algorithm is capable of efficiently factoring large numbers, a task that is considered computationally difficult for classical computers. By efficiently solving this problem, Shor’s Algorithm has the potential to break traditional cryptographic systems, such as RSA encryption, which rely on the difficulty of factorizing large numbers for security.
On the other hand, Grover’s Algorithm, proposed by Lov Grover in 1996, addresses the issue of searching unsorted databases. Unlike classical algorithms that require a time complexity of O(n), Grover’s Algorithm achieves a quadratic speedup, reducing the time complexity to O(√n). This quantum algorithm is particularly promising for optimization problems and has the capability to provide a significant speedup compared to classical algorithms in various search-related tasks, making it a valuable tool for future quantum computing applications.
Quantum Computing Hardware: Quantum Gates and Quantum Circuits
In the realm of quantum computing hardware, quantum gates and quantum circuits are fundamental components that govern the manipulation of information at the quantum level. Quantum gates are analogous to classical logic gates but operate on qubits, the quantum counterpart of classical bits. These gates perform operations such as flipping the qubit state or creating entanglement between qubits, paving the way for complex quantum algorithms to be executed.
Quantum circuits are constructed by connecting various quantum gates in a specific sequence to perform a desired quantum computation. These circuits showcase the flow of information within a quantum algorithm, demonstrating how qubits evolve and interact through successive gate operations. By designing efficient quantum circuits, researchers aim to optimize the performance of quantum algorithms and ultimately unlock the full potential of quantum computing technology.
Challenges in Quantum Computing: Error Correction and Decoherence
Quantum computing faces significant challenges due to the delicate nature of quantum systems. One major hurdle in developing practical quantum computers is error correction. As quantum bits, or qubits, are highly susceptible to errors caused by external interference, maintaining the integrity of the quantum information is crucial for the reliability of quantum computations. Developing efficient error correction codes that can effectively detect and correct errors without compromising the speed and power of quantum computing is a key area of research in the field.
Another challenge that quantum computing must overcome is decoherence. Decoherence refers to the loss of quantum coherence in a system, leading to the collapse of quantum states and the loss of quantum information. Preventing decoherence and preserving quantum superposition and entanglement over extended periods of time are essential for the successful functioning of quantum computers. Efforts to mitigate the effects of decoherence through advanced error correction techniques and innovative hardware designs are critical to realizing the full potential of quantum computing.
Applications of Quantum Computing: Cryptography, Optimization, and Simulation
Quantum computing holds immense promise in the realm of cryptography, offering capabilities that surpass what classical computers can achieve. Its ability to efficiently factor large numbers and solve complex mathematical problems make current encryption methods vulnerable to quantum attacks obsolete. By leveraging quantum principles, such as superposition and entanglement, quantum computers have the potential to revolutionize the field of cryptography, paving the way for unbreakable encryption and enhanced data security.
Moreover, quantum computing is poised to revolutionize optimization problems in various fields, ranging from finance to logistics. The inherent parallelism of quantum algorithms enables them to swiftly navigate through vast solution spaces, facilitating quicker and more accurate optimization processes. This can lead to significant advancements in resource allocation, supply chain management, and portfolio optimization, enhancing decision-making processes and driving efficiency across industries. Additionally, quantum simulation promises to revolutionize scientific research by enabling the accurate modeling and simulation of complex systems that are beyond the capabilities of classical computers, providing valuable insights into intricate phenomena across disciplines.
The Future of Quantum Computing: Potential Impact on Society and Technology
Quantum computing has the potential to revolutionize various aspects of society and technology in the future. With the ability to solve complex problems at an exponential speed compared to classical computers, quantum computing could accelerate advancements in fields such as drug discovery, material science, and artificial intelligence. This could lead to significant breakthroughs in medical research, sustainable energy development, and the optimization of logistical and financial processes.
Furthermore, the impact of quantum computing on data security and encryption cannot be understated. Quantum computers have the capability to break traditional cryptographic methods, which calls for the development of new quantum-resistant algorithms to safeguard sensitive information. As quantum computing continues to progress, it will be crucial for organizations and governments to adapt and enhance their cybersecurity measures to protect against potential threats posed by quantum-powered attacks.
Leave a Reply